Axions: leading candidate for dark matter or not?
- Kate Green
- Oct 19, 2024
- 9 min read
Updated: Nov 11, 2024
by Kate Green
October 19, 2024
A collaborative team of physicists from the universities of Amsterdam, Princeton, and Oxford has recently published findings suggesting that extremely light particles known as axions may form large clouds around neutron stars. These axions offer a potential explanation for dark matter, a phenomenon that has long eluded cosmologists. Importantly, the research indicates that these axion clouds might be observable with existing telescopes.
Background on Axions
Previous Research:
The team previously explored axions that escape neutron stars, investigating how they could lead to observable signals when converted into light under certain conditions.
Current Focus:
The latest research shifts attention to axions that do not escape the gravitational pull of neutron stars, focusing on those captured and retained by the star’s immense gravity.
Formation of Axion Clouds
Capturing Axions:
Over time, axions that are gravitationally bound to neutron stars accumulate, gradually forming a dense, hazy cloud around the star.
Observational Potential:
The formation of these axion clouds raises intriguing questions for astronomers and physicists alike. The existence of such clouds could lead to observable phenomena that might provide insights into both axion physics and the nature of dark matter.
Why Study Axion Clouds?
Exploration of Dark Matter:
Understanding axion clouds could help elucidate the properties and behaviours of dark matter, contributing to a more comprehensive understanding of the universe’s composition.
Observable Signals:
The clouds may produce detectable signals that can be studied using current astronomical instruments, enabling researchers to test theories regarding axions and dark matter.
Link to Neutron Stars:
Neutron stars represent extreme environments, making them ideal laboratories for studying the fundamental interactions of particles like axions. Observing these clouds could yield valuable data about the physics governing both neutron stars and potential dark matter candidates.
The study of axion clouds around neutron stars presents a promising avenue for advancing our understanding of dark matter. By focusing on the axions retained by these stars, physicists aim to uncover observable signals that may confirm the existence of axions and provide critical insights into the nature of the universe. This research exemplifies the interconnectedness of particle physics, astrophysics, and cosmology, as scientists strive to unravel one of the most profound mysteries of modern physics.
Axions: From soap to dark matter
Introduction to Axions
Understanding Common Particles:
Many people are familiar with protons, neutrons, electrons, and photons—fundamental particles that constitute the matter around us. In contrast, axions are less known and remain a theoretical entity.
Hypothetical Nature:
Axions are currently only a hypothetical type of particle; no experimental evidence has yet confirmed their existence. Their conception emerged from the need to address theoretical inconsistencies within particle physics.
Origin and Naming
Naming and Historical Context:
Proposed in the 1970s, the term “axion” was inspired by a brand of soap, reflecting its role in “cleaning up” theoretical problems concerning the strong interactions of particles like the neutron.
Theoretical Framework:
In particular, axions were introduced as part of the solution to the strong CP problem, which involves the apparent absence of certain types of symmetry violations in strong interactions. Their introduction aimed to reconcile theoretical predictions with observed phenomena.
Characteristics of Axions
Hypothetical Nature:
Despite being a compelling theoretical candidate, axions have not yet been detected. They are predicted to be extremely light—much lighter than protons, neutrons, or electrons—making them difficult to observe directly in laboratory experiments.
Weak Interactions:
Axions are theorized to have very weak interactions with other particles, meaning that even if they do exist, they would be challenging to detect with existing experimental setups. This property makes them intriguing candidates for dark matter.
Axions and Dark Matter
If axions exist, they could potentially account for a significant portion of the universe's dark matter due to their ability to cluster and exert gravitational influence on galaxies and cosmic structures.
Dark Matter Overview:
Approximately 85% of the universe's matter content is thought to be "dark matter," which interacts with normal matter primarily through gravitational forces. This dark matter does not emit, absorb, or reflect light, making it invisible and detectable only through its gravitational effects on visible matter, such as galaxies and galaxy clusters.
Detection Challenges:
The notion that axions could contribute to dark matter has gained traction, as they may account for the unseen mass influencing the universe's structure and behaviour.
Despite the theoretical implications, dark matter interactions with normal matter are presumed to be minimal, making it exceptionally difficult to detect.
The axion remains one of the most intriguing candidates for solving the dark matter mystery, bridging gaps between fundamental physics and the cosmos. Thus, the quest to detect axions continues, with physicists exploring innovative experimental approaches to uncover the nature of dark matter and the universe's composition. If proven to exist, axions could illuminate our understanding of the universe and provide answers to some of the most profound questions in modern physics. The theoretical possibility that axions could be a key component of dark matter emphasizes the intricate connections between particle physics, cosmology, and our understanding of the universe.
Neutron stars as magnifying glasses
The concept of neutron stars acting as “magnifying glasses” for axions is a fascinating approach to addressing the challenge of detecting these elusive particles.
Here is a deeper dive into how this works and its implications for axion research:
The Axion-Photon Conversion Mechanism
Electric and Magnetic Fields:
Axions are hypothesized to have the ability to convert into photons (light particles) when subjected to electric and magnetic fields. This conversion is a crucial aspect of their potential detectability.
However, the interaction strength between axions and photons is expected to be extremely weak, meaning that the amount of light produced from axions under typical conditions would be minimal.
Conditions in Neutron Stars:
Neutron stars provide a unique environment due to their extreme density and strong gravitational fields. These stars are typically around 12 to 15 kilometres in radius but contain masses similar to that of the Sun, creating immense pressure and temperature.
The magnetic fields around neutron stars can be billions of times stronger than those on Earth, offering an ideal setting for the mass production of axions. Under these conditions, the density of axions could become substantial enough to enhance the likelihood of photon production.
The Potential for Observable Signals
Enhanced Signal Detection:
The dense clouds of axions formed around neutron stars increase the chance of axion-photon conversion events, leading to potentially observable signals.
If a significant number of axions can convert into photons due to the extreme magnetic fields present in neutron stars, it may produce a detectable light signature that astronomers can observe.
Types of Signals:
Continuous Emission: During the neutron star's active phase, a steady emission of light could be produced as axions convert to photons. This signal may be detectable over long periods.
Transient Events: A dramatic one-time burst of light could occur at the end of a neutron star's life, potentially offering a clear and unique signal that could be studied in detail.
Broader Implications for Axion Research
Testing Dark Matter Theories:
Observing these axion-induced signals would provide valuable data that could either support or challenge current models of dark matter. If axions are confirmed to exist and are observed through these mechanisms, it would significantly advance our understanding of the universe’s composition.
Increased Collaboration:
The study of axions in neutron stars could lead to cross-disciplinary collaborations between astrophysicists, particle physicists, and experimental scientists as they work together to develop and deploy observational strategies.
Future Research Directions:
Continued theoretical and computational modelling will be essential to refine predictions about axion clouds and their potential signals. Additionally, the development of new observational techniques and technologies will enhance the search for these elusive particles.
Neutron stars, with their extreme conditions, present a promising avenue for detecting axions and addressing fundamental questions about dark matter. By leveraging the unique properties of these stellar remnants, researchers are better positioned to uncover new physics and enhance our understanding of the universe. The potential to observe axion-induced light from neutron stars not only offers hope for confirming the existence of these hypothetical particles but also for unravelling the mysteries of dark matter that have puzzled scientists for decades.
The ones that stay behind
The authors’ research marks a significant advancement in our understanding of axions, particularly focusing on those that are captured by neutron stars rather than escaping into space. Here is a detailed breakdown of their findings and implications:
Key Findings
Axion Capture:
The study shifts the focus from axions that escape neutron stars to those that are gravitationally bound to them. These captured axions, despite their extremely low mass and weak interactions, can accumulate around the neutron star over millions of years.
Formation of Axion Clouds:
The authors argue that axion clouds should exist around most neutron stars due to their gravitational pull, leading to the formation of dense axion clouds. These clouds may be significantly denser—potentially up to twenty orders of magnitude—than local dark matter densities, presenting a unique opportunity for observation.
Observational Signatures
The dense axion clouds around neutron stars could produce various observable signals, primarily:
Continuous Signals: Emitted over extended periods during the neutron star's lifetime, providing a steady light source for telescopes to detect.
One-Time Bursts: Occurring at the end of a neutron star's life when it ceases its electromagnetic emissions. This burst could serve as a distinct signal for axion detection.
Implications for Axion Research
Research Opportunities
The presence of axion clouds around neutron stars creates exciting avenues for research. These clouds can be studied to probe the properties and interactions of axions, contributing to our understanding of dark matter and fundamental particle physics.
Utilization of Existing Telescopes:
The potential for detection using existing radio telescopes is significant, as it allows for immediate observational efforts without the need for new, complex instrumentation. This accessibility could expedite the search for evidence of axions.
Understanding Neutron Star Dynamics:
The formation and characteristics of axion clouds could also provide insights into the dynamics and evolution of neutron stars, enriching our knowledge of stellar physics.
The authors’ focus on axion clouds around neutron stars opens up a promising frontier in astrophysics. By studying these clouds, researchers may not only advance our understanding of axions as a candidate for dark matter but also enhance the broader understanding of neutron star physics and cosmic structure. The potential to observe distinct signals from axion clouds could lead to groundbreaking discoveries, making this a highly impactful area of research in the ongoing quest to unravel the mysteries of the universe.
What is next?
The next steps in the study of axion clouds around neutron stars are multifaceted and aim to deepen our understanding of both axions and neutron stars themselves. Here are some key directions for future research:
Search for Axion Clouds
Observational Campaigns: The primary focus will be on conducting thorough searches for axion clouds using existing radio telescopes and observatories. Scientists will look for the predicted signals associated with axion emissions, both continuous and one-time bursts, to confirm their existence.
Theoretical Investigations
Dynamics of Neutron Stars: One of the authors is already working on exploring how the presence of axion clouds could influence the dynamics of neutron stars. Understanding these interactions could provide insights into the internal structure and evolution of these dense objects.
Numerical Modelling
Refining Predictions: More numerical modelling of axion clouds is essential to provide accurate predictions of their formation, behaviour, and the resulting observational signatures. This modelling will help researchers identify specific parameters to focus on during observations.
Binaries and Axion Clouds
Studying Binaries: Many neutron stars exist in binary systems, either with another neutron star or with a black hole. Investigating how axion clouds behave in these environments will be critical. Researchers will need to understand how the dynamics of axion clouds might change in these complex systems and what unique signals they could produce.
Cross-Disciplinary Collaborations
Integrative Research: As the study of axions intersects with various fields—particle physics, astrophysics, plasma physics, and observational astronomy—collaborations among experts in these disciplines will be important. This integrative approach can lead to new methodologies and enhance the overall understanding of dark matter and fundamental physics.
Future Theoretical Developments
Exploring Alternative Scenarios: Researchers might explore alternative theories and scenarios involving axions and their potential role in the broader context of cosmology, dark matter, and the evolution of the universe.
The exciting prospect of discovering axion clouds represents a significant step in understanding dark matter and the fundamental forces governing the universe. By establishing a comprehensive research agenda focused on both observational and theoretical advancements, scientists hope to illuminate the mysteries surrounding these elusive particles and their implications for astrophysics. As this field progresses, it may reveal profound insights into the nature of matter and the structure of the cosmos.
Journal:
Dion Noordhuis et al, Axion Clouds around Neutron Stars, Physical Review X (2024). DOI: 10.1103/PhysRevX.14.041015
Image:
An axion cloud around a neutron star. While some axions escape the star’s gravitational pull, many remain bound to the star and over a long period of time form a cloud surrounding it. The interaction with the neutron star’s strong magnetic field causes some axions to convert into photons – light that we can eventually detect with our telescopes on Earth. Credit: University of Amsterdam
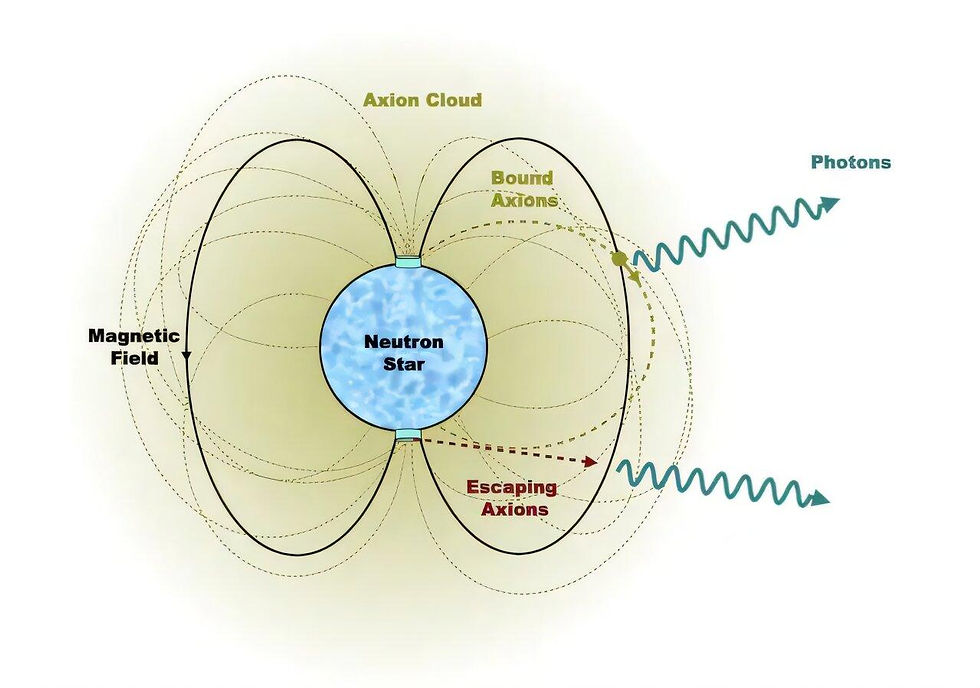
Image:
Overview of the four stages characterizing the formation and evolution of axion clouds around neutron stars. Credit: Physical Review X (2024). DOI: 10.1103/PhysRevX.14.041015
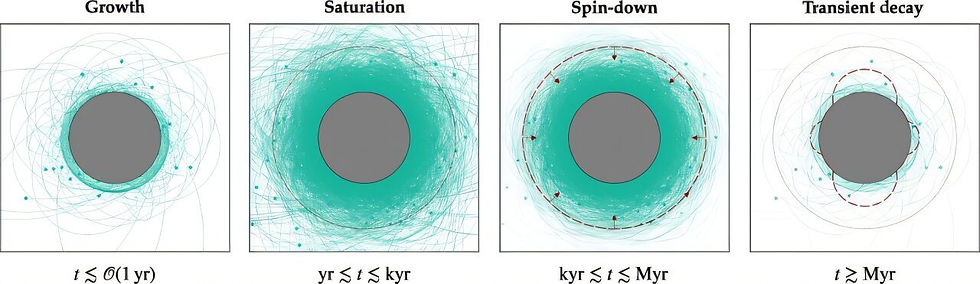
Comentarios