First results from the Axion Dark-Matter Birefringent Cavity experiment establish a new technique for axion search
- Kate Green
- Oct 20, 2024
- 4 min read
Updated: Nov 11, 2024
by Kate Green
October 20, 2024
MIT researchers recently published the first results of an experiment aimed at detecting axion dark matter by exploring axion-induced birefringence of electromagnetic waves. Although no axion signals were observed, the findings, published in Physical Review Letters, introduced a new method for axion searches. This approach uses a tuneable optical cavity, allowing researchers to adjust the experimental parameters and expand the range of possible axion masses they can probe. This marks a significant advancement in the search for axions, hypothetical particles thought to be a key component of dark matter.
Evan Hall, a research scientist at MIT's LIGO lab, explained that the experiment was first proposed in 2019 through a collaboration between their lab and the MIT Center for Theoretical Physics. The team was exploring new approaches to search for the axion, a hypothetical dark matter particle.
"Any observable interaction between axions and standard matter is expected to be very faint. We realized that the problem of trying to find a faint signal was a very similar problem to the detection of gravitational waves, which is another kind of very faint kind of signal detected only recently" Hall stated.
After extensive theoretical discussions, Evan Hall and his colleagues recognized that the lasers and optical instruments used in LIGO's gravitational wave detection could be adapted for axion searches. This led to the development of the Axion Dark-Matter Birefringent Cavity (ADBC) experiment, which began collecting data in 2022. The experiment repurposes LIGO’s technology to probe for faint signals that could reveal the existence of axions, a type of hypothetical dark matter particle.
"We wanted to demonstrate experimentally how to use these tools to pull off an axion search," explained Hall. "Light comes in two polarizations—horizontal and vertical. Axions, if they exist, are expected to convert one polarization into the other. In our lab, we use a laser to generate vertically polarized light, and we look for any hint that axions have converted some of that light into the horizontal polarization."
The ADBC experiment's detector is composed of four mirrors arranged in an optical cavity, a structure designed to trap and recirculate light. This cavity allows laser-generated light beams to bounce thousands of times, significantly amplifying any faint signals that could be caused by axions. This design enhances the experiment's ability to detect the subtle interactions expected from these hypothetical dark matter particles.
In the early stages of the experiment, Hall and his team used the ADBC detector to search for axions with masses around 50 neV (about 10–40 grams). Interestingly, a research group in the United Kingdom has been conducting similar searches for axions, focusing on those with masses around 2 neV, using equipment comparable to that used at MIT. This highlights the growing global interest in axion searches, with different teams probing various mass ranges to uncover these elusive dark matter particles.
"Our work showed that this new kind of cavity can be tuned to widen the range of possible axion masses that can be searched over," said Swadha Pandey, fourth year Ph.D. student at MIT. "Specifically, we showed that the cavity can be tuned by adjusting the angles of its four mirrors. Tunability is an important criterion for making the apparatus useful for dark matter searches. Since nobody knows what the axion mass might be, we need to search over a wide range of possible masses."
The initial results from the ADBC experiment have placed important constraints on the potential coupling between axion-like particles and photons. While axions have not been detected yet, these findings lay the groundwork for future research, showing that optical cavities can be an effective tool for searching for these hypothetical dark matter particles. This work could inspire further experiments and refinements in the search for axions, bringing researchers closer to their potential experimental discovery.
"Having shown that this method can work over a broad range of axion masses, the next step would be to build a larger, more sensitive experiment," added Pandey. "Such an experiment would use more laser light, so that there are more photons interacting with the axions, and the experiment would be larger, to increase the time that the photons can interact with the axions. Automation of the tuning mechanism and clever designs for mirror coatings are also essential for scanning across the entire available range of axion masses."
Journal:
Swadha Pandey et al, First Results from the Axion Dark-Matter Birefringent Cavity (ADBC) Experiment, Physical Review Letters (2024).
DOI: 10.1103/PhysRevLett.133.111003
Image:
Experimental setup at MIT, picture by Swadha Pandey. Our four-mirror detector is housed inside two steel vacuum chambers connected by a pipe. The platform in front of the near vacuum chamber supports optics that direct laser light into and out of the chamber. Credit: Pandey, Hall and Evans.
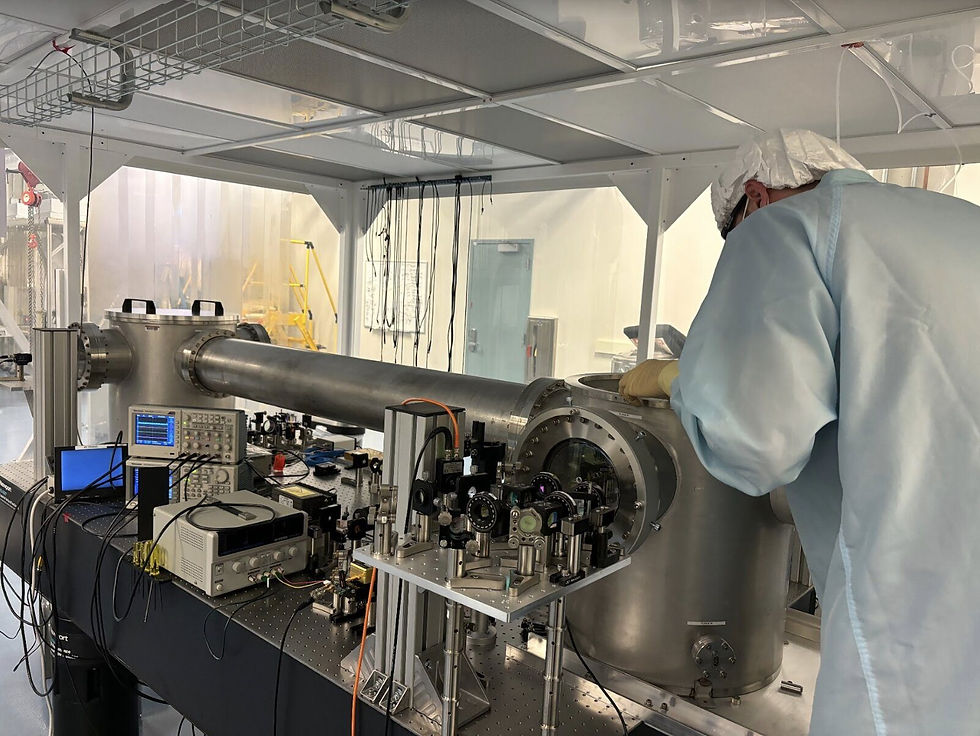
Image:
Experimental setup: bow-tie cavity with A, D, B, C mirrors. ^𝑠-polarized pump field from a 1064 nm Nd:YAG laser enters the cavity at mirror A and is locked to the cavity using a Pound-Drever-Hall lock. ^𝑝-polarized sidebands generated by an axionlike particle (ALP) at the cavity splitting frequency 𝜔𝑎=𝜔sp are resonant in the cavity. Heterodyne readout is performed using pump and signal field transmitted at mirror C. Credit: Physical Review Letters (2024). DOI: 10.1103/PhysRevLett.133.111003

Comentarios